Accurate Network Design Needed to Harness the Full Potential of Metamaterials
Share
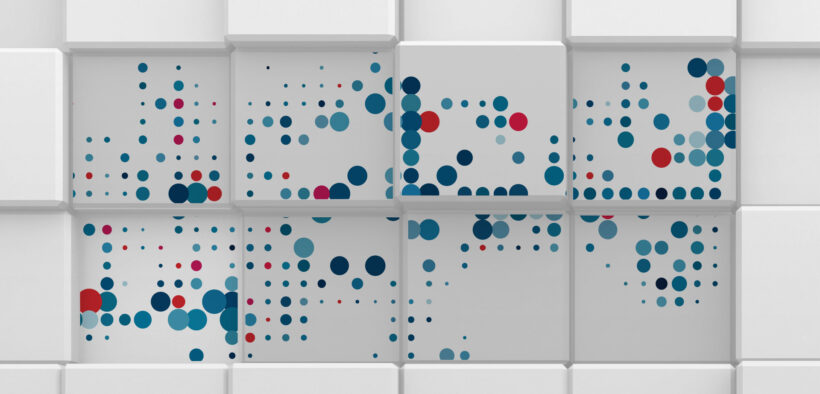
More coverage at less cost is the holy grail of in-building wireless network design. Whether you’re deploying an LTE network or a 5G network at 28 GHz or 3.5 GHz, the whole point of going through the site survey, prediction modeling, and design effort is to determine the ideal placement of antennas and access points to deliver the optimal coverage for an enclosed space. This overarching objective holds true for any in-building network design, whether it is for a large, multi-floor deployment in a downtown office building, a warehouse distribution center at the edge of town, or a multi-floor shopping mall.
The ideal design eliminates the risk of over-designing or under-designing the network, thereby enabling a more efficient deployment that provides the coverage needed. To date, the key variables that network designers have had to take into consideration to ensure antennas and access points provide that coverage have been the size of the space, the number of floors, the configuration of the coverage area, obstructions that could affect signal propagation, potential interference, and dead zones.
But ongoing research and development efforts with metamaterials and metasurfaces may soon throw more variables into the mix. As they move from research labs and proof of concept into widespread application, the importance of accurate prediction and design will become even more critical to providing the coverage needed to deliver high-quality, seamless user experiences cost-effectively in indoor spaces.
Engineered Materials Enable Signal Manipulation
Metamaterials have been a hot topic in microwave and RF circles for at least 20 years, primarily because of potential applications in wireless networks. These artificially engineered materials are built with microstructures known as “meta-atoms,” which are much smaller than the wavelength of an electromagnetic wave and have electromagnetic properties that are not found in naturally occurring materials.
Most metamaterial research is focused on creating Negative Index Materials (NIMs) that have a negative refraction index (NRI). These materials refract electromagnetic waves in a different direction compared to conventional materials with positive refractive properties. As a result, metamaterials with an NRI can be used to control and manipulate electromagnetic waves.
To date, metamaterials have been used in the design and construction of RF and microwave antennas to make them smaller and more powerful. But it’s their potential use as metasurfaces in construction materials for buildings that may soon affect how indoor wireless networks are designed.
More Potential Options for Signal Propagation
Research into the future applications of metamaterials points to significant potential in the creation of reconfigurable intelligent surfaces (RISs). An RIS is a thin surface composed of multiple small antennas that have been created with NIMs to receive and passively re-radiate an RF or microwave signal.
In its simplest form, an RIS can be a dynamic reflectarray built with multiple omnidirectional antennas. A more elaborate implementation would be to use an RIS as a dynamically tunable metasurface, which can not only scatter and phase-shift a signal but can also have a controllable reflection angle and even polarization manipulation abilities.
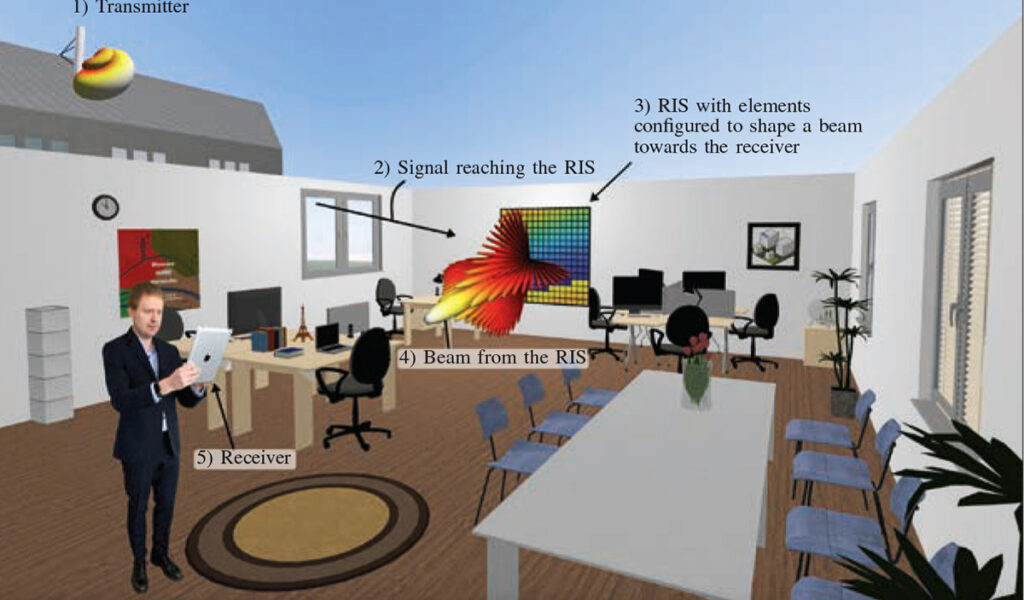
With an RIS, wireless signals could be altered in ways not possible with traditional MIMO arrays. The RIS could be used to keep the wireless channel well-conditioned, which increases the achievable data rate. It could also be used to mitigate the effects of Doppler spread and multipath fading. And in the future, RISs could be applied to make the use of TeraHerz signals a reality.
In short, by leveraging the full potential of metamaterials, it may soon be possible to integrate RISs in the construction of walls and ceilings to improve network coverage in office buildings, warehouses, and even shopping malls in areas where today’s antennas aren’t effective. Strategic positioning of RISs can ensure optimal coverage in dead zones, around obstructions, and even around corners.
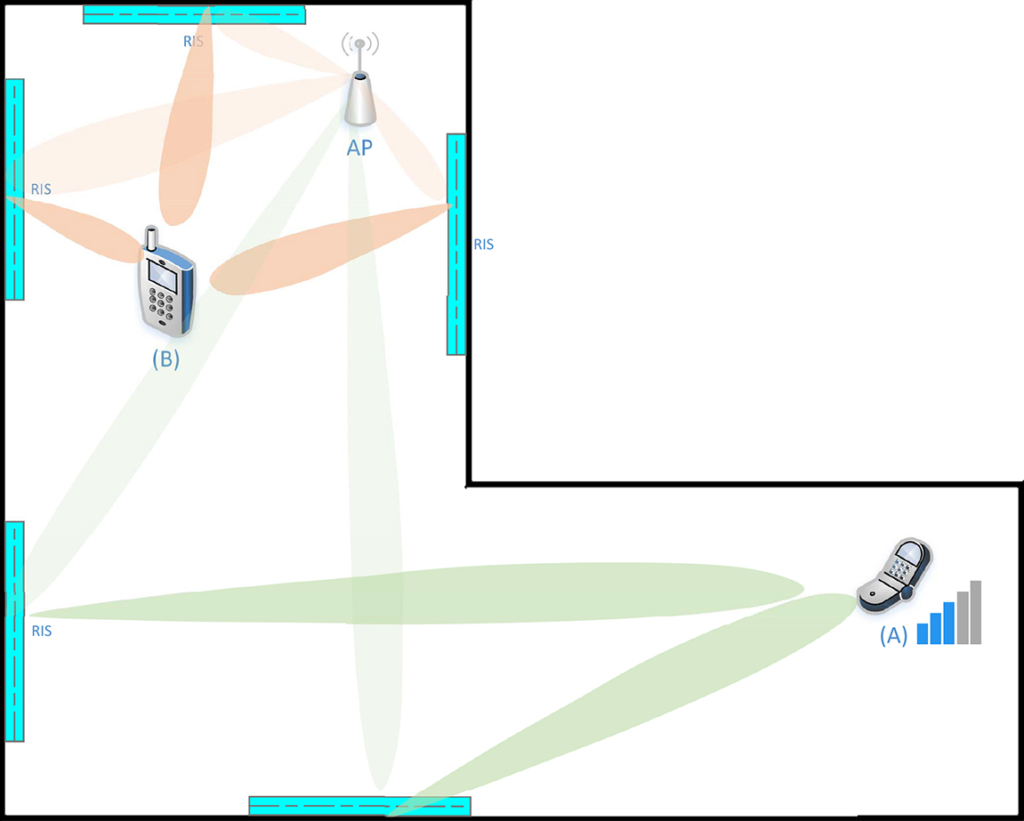
New Solutions Also Create New Challenges
Of course, while more propagation options can help solve many of today’s challenges with in-building network design, they can also create new challenges.
As noted in our metamaterials white paper, strict modeling of reflective metasurfaces requires full wave analysis, which is costly in terms of CPU usage. But an alternative hybrid method can be used. This method is a combination of ray tracing applied everywhere but on RIS, and a full wave analysis applied on RIS. It requires full wave simulation to get the complex radar cross section (RCS) of the RIS. The complex RCS is used to represent the RIS as a secondary radiation source in the ray traces. The final signal (electric field) distribution in the network is a summation of two field components: one due to the presence of the RCS/RIS, and the another approximated by a ray tracing algorithm, which would exist if the RIS had not been implemented.
Accurate Prediction and Design Required
As the potential uses of RISs emerge, the ability of network designers to accurately predict and design a network before deployment will be more critical than ever. Full wave electromagnetic simulation will be needed to fully capture the interaction of an incoming wave with an RIS and compute the reflected fields both in the near and the far field of the surfaces.
However, ray tracing, an asymptotic method that is widely used for propagation modeling, seems to be the most efficient way for designers to simulate and model coverage for any space, in terms of CPU usage versus prediction accuracy.
iBwave provides the right combination of elements needed to streamline the design of all indoor wireless networks and is working to support modeling of networks that leverage the capabilities of RISs. To enable accurate planning and design, iBwave Design software offers three prediction models — VPLE, COST 231, and Fast Ray Tracing — that provide different levels of accuracy for different environments. Most of our customers rely on the Fast Ray Tracing prediction algorithm, which is based on Ray Tracing and was developed by iBwave RF engineers in partnership with scholars and experts from the in-building industry.
Read the metamaterials white paper to learn more about metamaterials, RISs, and how prediction modeling based on ray tracing can be used to streamline the design of today’s and tomorrow’s in-building networks.
- At a Glance: What Is New in Wi-Fi 7? - July 4, 2024
- Accurate Prediction Simplifies Private, In-Building 5G Network Deployments - March 1, 2023
- Accurate Network Design Needed to Harness the Full Potential of Metamaterials - February 20, 2023
The RIS’s in the telecommunications of RF materials seem to be in opposition to accurate prediction and design. The beam from Tera Hertz signals to the AP create an electromagnetic tension that may be shielded from the building structure. I’m just saying. Sorry for the minimalist comment.